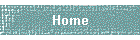
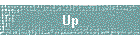
| | Here are some notes about systems theory - the idea that different systems have properties in common with each other. Biological systems are 'open systems' since they continuously take in matter, energy, and information, and continuously pass materials, energy, and information to their environment. Summary An open system may be visualised as having an incomplete boundary through which selected matter, energy, and information can enter (‘inputs’) and different matter, energy, and information can leave (‘outputs’). Within the system, metabolic processes link inputs with outputs. Open systems interact to form complex networks with hierarchical forms and at some point may be considered to ‘emerge’ at the next level of organisation. For example, molecules organised in a particular way emerge as cells. It is then hypothesised that most or all open systems at each level have the capacity to create an internal ‘model’ of some kind. A visual representation based on the idea of a fractal network is provided to illustrate how models at different levels of organisation might interact with each other. 
| An open system Wherever there is a ‘thing’, there is a partial separation between it and everything else, some kind of boundary (Figure 1). | 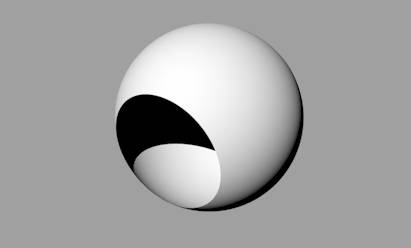 Figure 1: The boundary of an open system is represented as a ‘shell’ with an opening | 
| It is a separation that allows things to happen and differences to exist which might not otherwise occur. Some boundaries have a physical reality such as the plasma membrane of a cell or the skin, but other boundaries may be more organisational than physical and determined to a degree by the observer (Figure 2). | 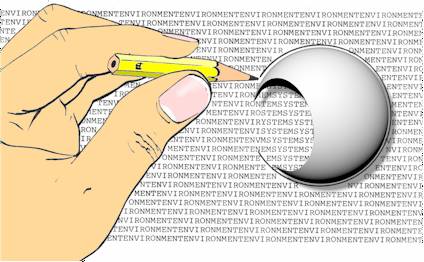 Figure 2: Some boundaries are drawn by the observer rather than having a physical reality | 
| Boundaries are never complete, and selected matter, energy, and information can enter and leave the open system which is only partially separated from everything else. The inputs and outputs are linked by the metabolic processes going on within the system (Figure 3). | 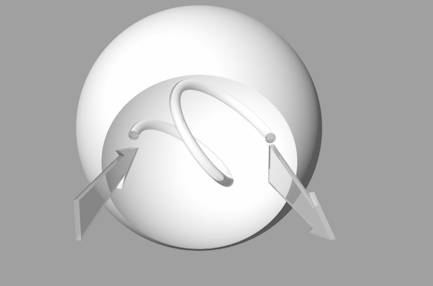 Figure 3: An open system with inputs, metabolic processes (represented by the spiral shape), and outputs | 
| Interactions between open systems If an output of an open system is of some use to another nearby open system, then we can envisage the systems moving closer together to form a mutually beneficial reciprocal relationship (Figure 4). | 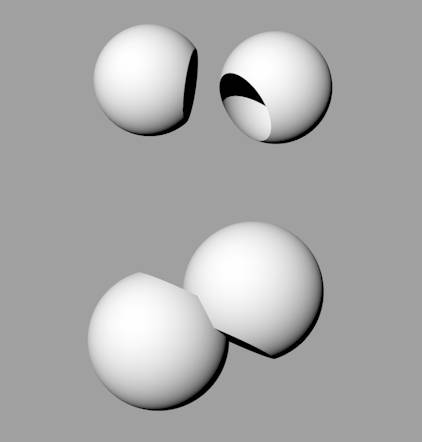 Figure 4: Two open systems coming into a mutually-beneficial reciprocal relationship | 
| As systems cluster together, their organisation becomes more complex. Some systems might become more centrally placed within a cluster, interacting directly with many other systems, in which case they would have more influence over the activities of neighbouring systems than a system perched out on the rim with fewer connections. To indicate these differing degrees of influence within a cluster the systems can be drawn at different relative sizes – the more central and more influential members of the group can be shown larger, while the more peripheral and less influential systems are drawn smaller – size is being used to indicate relative power within the group. We are beginning to see the emergence of hierarchy within the cluster (Figure 5). There are power differentials within the network of interactions between member systems. More central means more powerful – promotion means moving in towards the centre. | 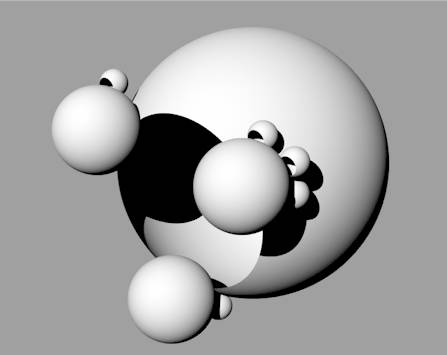 Figure 5: A hierarchical cluster of open systems | 
| Within such a cluster, a new type of interaction between systems becomes worthwhile: similar systems with similar needs can work together as a coalition to increase their relative power within the cluster (Figure 6). We can imagine that the systems illustrated so far are different types of atoms coming together and combining in different ways to form molecules. Some molecules are unstable and transient, others are longer lived. Some are simple, some are very complex. Thus all the events have been occurring at one level of organisation, in this case the atomic level. Let’s call this level 1, a completely arbitrary label to start from and is not meant to imply that atoms form the lowest level of organisation, which of course they do not. | 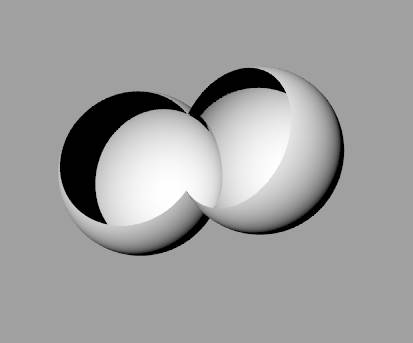
Figure 6: Two open systems forming a coalition | 
| Emergence at a new level of organisation At some point, the evolving complexity of some of the molecular clusters and their interactions becomes so great that something remarkable happens – a living cell is formed. We have no clear idea about how this happens, but the individual systems (molecules) from which the cell is made contribute to the cell’s ability to move around, react to the environment, and reproduce. This quantum leap, the emergence of a new type of system, can be modelled by creating a new level of organisation - level 2 - ‘above’ level 1. If we stick with the example chosen, then level 2 is the cellular level. Even though the cells are all made of atoms and molecules that inhabit level 1, the behaviour of each cell can be considered in its own right, without having to describe it only in terms of chemical interactions. The cell exhibits ‘wholeness’ at the new level of organisation. Thus we can draw a new (open) boundary at level 2 around all the systems at level 1 which make up the cell. | 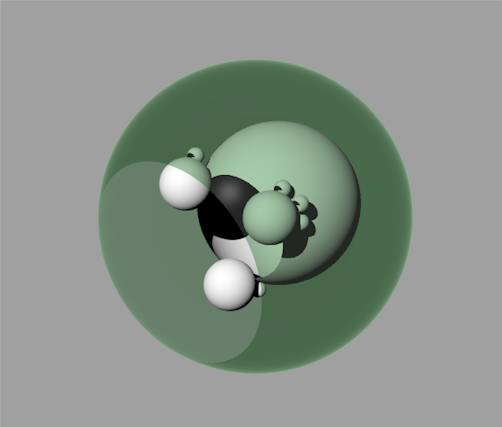 Figure 7: The outer shell represents the boundary of the system (built from systems at level 1) that has emerged at level 2 | 
| If we now model level 2, we can visualise similar things happening to those at level 1 – some systems (cells) clustering together in reciprocal and coalition relationships, the emergence of hierarchy and so on. In the abstract world we are creating, the rules are the same at each level of organisation. At some point, clusters of cells become so exquisitely organised that they form organisms which emerge at a new level of organisation, level 3. A new boundary is created at that level. By now the process of emergence is becoming more familiar, so we can imagine organisms interacting together to form societies (level 4), and societies interacting together to form the United Nations (level 5). | 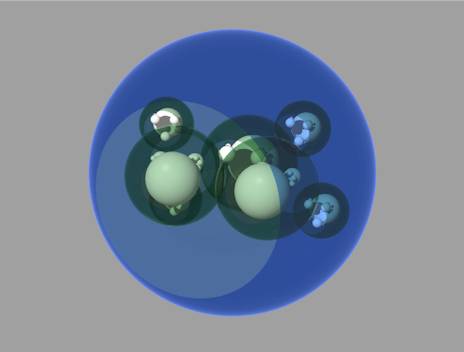 Figure 8: Emergence at level 3 | 
| Modelling We, as observers, model the world in which we live. We form a world view that continues to evolve as we gain experience throughout our lives. It is not clear to what extent other systems make internal representational ‘models’. Since all open systems are composed of a selected sample of matter, energy, and/or information that originally was ‘out there’ in the environment, it can be suggested that by their very existence they form something of a ‘memory’ of an earlier time in the evolution of the environment. Clearly, many open systems, especially the more complex ones such as living cells and organisms, are more than just a passive repository of earlier states. They process information from the environment, react to it, change their behaviour through experience, and possibly plan for future actions. They develop internal representations that help in their survival. This means that a new element can be added to our little egg-shell representation of an open system – a modelling capacity (Figure 9). | 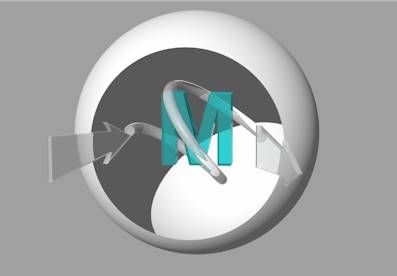
Figure 9: An open system has the capacity to form an internal representation or model (‘M’) | 
| No attempt will be made to pin down the exact meaning of 'model' at this stage; rather, it will be used to stand for representational activities in general, sentient or otherwise. Therefore, at the human level, 'model' would include both conscious and subconscious activities. Thus, modelling capacities can be added at every level of our abstract universe – at lower levels the capacity is small, at higher levels the capacity is more sophisticated. The possession of a model means that the behaviour of a system, particularly a complex system, is not mechanical and predictable since the model influences future behaviour and provides the system with a degree of autonomy over its environment. At least, that would be the impression given to an observer, since stimulus-response loops will be buffered and modified by the computational activities of the model. The result will be that the system's responses show variation over time: the response elicited by a particular input pattern may not be the same when the same input is tried again after the system has gained other experiences in the interim. | 
| Linking models at different levels There is, however, a shortcoming in this way of looking at the world and the systems within it. The stratification of the observed world into levels of organization seems helpful enough in the sense that it recognizes the apparent ‘wholeness' of a molecule, cell, or person, and identifies repeating patterns at each level, but the problem then arises of understanding how events at one level impinge upon events at another. For example, do events at a higher level determine what happens at lower levels (‘top-down' causation), or do events at lower levels dictate what happens at higher levels ('bottom-up' causation)? Or can both occur? The problem of linkage also applies to the proposal that models may exist in systems at different levels: how would those models interact? For example, how would models within cells possibly interact with or in some other way contribute to consciousness at the human level (Figure 10)? Although the systems approach adopted so far has difficulty in suggesting how models at different levels might interact, it is possible to take the process forward by introducing the observed properties of neural networks. | 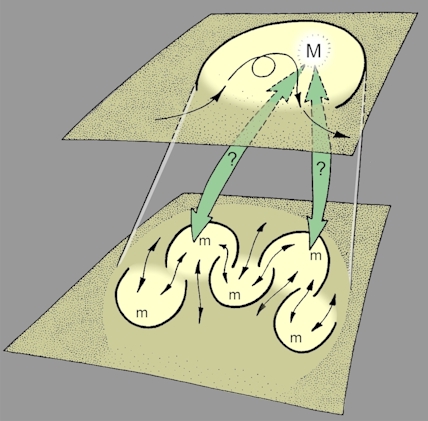
Figure 10: How do models at the lower level link with a metamodel at the next level? | 
| Neural networks The term 'neural network' is rather loosely used and may refer either to a network of biological neurons forming part of a nervous system (natural neural network) or a computer simulation of a network composed of interconnected units with neuron-like properties (artificial neural network). In both cases, each node in the network has one or several inputs of variable 'strength' (usually both excitatory and inhibitory) and summates the inputs, giving rise to an output or outputs when a stimulus threshold is crossed. Artificial neural networks are often simulated with three layers of units: an input layer, an intermediate 'hidden' layer, and an output layer (Figure 11). | 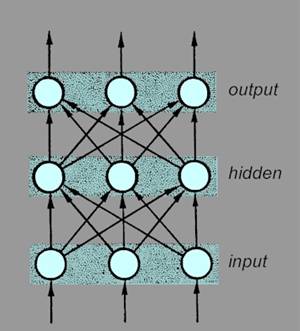
Figure 11: Arrangement of an artificial neural network with three layers of units | 
| The neural network is ‘trained’ to carry out a task by modifying the connections between the units. There are several algorithms that can be used for this purpose, the most studied being the back propagation method. This requires that the correct outcome is known before the task begins. Over repeated exposures to the task, connections that contribute towards correct behaviour become strengthened, while connections that contribute to aberrant behaviour are given less weight. As a result of perhaps thousands of trials, a distributed 'memory' of the task is established across the network in the pattern of different-strength connections. A trained network contains information in its pattern of connection strengths about associations and categories, and in the sense used above embodies a 'model' of its task (Figure 12). | 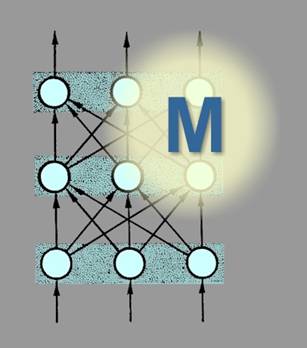
Figure 12: A trained network embodies a model (‘M’) of the task | 
| These studies have demonstrated that networks of interconnected units have the capacity to model, and this insight is helpful when considering other systems such as cells and parts of cells such as organelles and macromolecules. The units which form the nodes of a given artificial or natural neural network share most if not all of the properties already delineated for open systems: inputs, some kind of inner transformation or function, outputs, and interactions with other units. Therefore, it may be helpful to redraw a neural network with open systems as the units to make the link with the previous illustrations (Figure 13). | 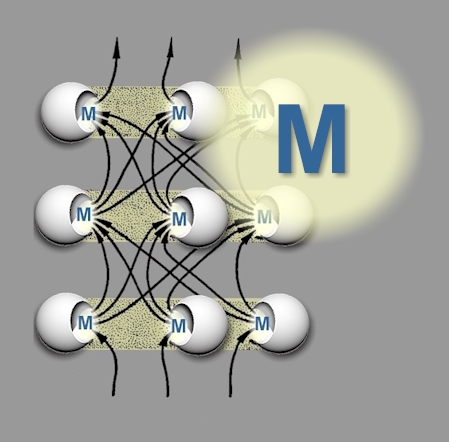
Figure 13: A neural network redrawn with the units represented as open systems (‘M’ = model) | 
| But by building on our experience with systems theory, we can take the analysis further. If we are considering a natural neural network, then neurons take the place of the units. Each neuron is a complex and living assemblage of interacting parts, and those parts can also be viewed as open systems. So we could model the neuron itself as a network with, for example, its organelles forming the units. Similarly, we could model organelles such as the mitochondria as networks, with their constituent molecules forming the units. This process of identifying network characteristics could probably be extended further to include molecules such as proteins which are known to respond to environmental cues. We begin to see an interconnected pattern of networks within networks. 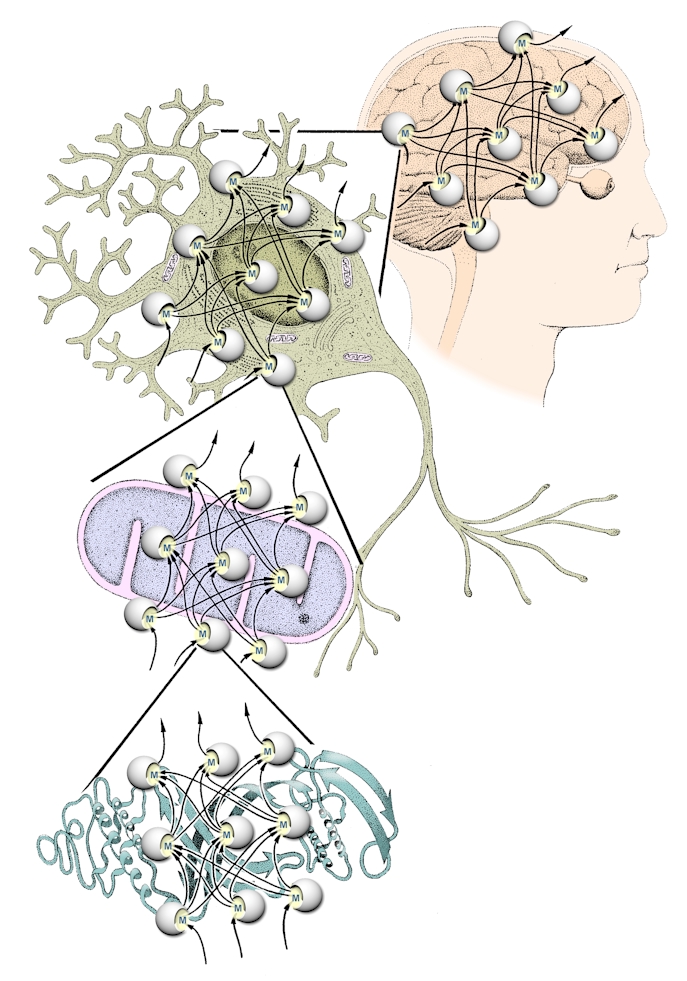
| 
| Looked at in this way, biological organization takes on the appearance of nested sets of networks, with the units in a network at one level being networks in their own right at the next level down, and the units at that lower level are also formed from networks, and so on. Each unit has a modelling capacity, is made up of a network of modelling units, and contributes to another network of modelling units that together contribute to the next higher level. A suitable term for this arrangement would be ‘fractal network’, since it captures the quality of self-similarity or recurring patterns at different levels - nets-within-nets. With the arrangement described here, links can be seen between modelling processes at different levels of organisation. If we consider for example one neuron within the brain, its behaviour within the network of interconnected brain neurons will depend not only on inputs received from other neurons forming synapses with it, but also on the activities within the network that makes up the neuron, including its modelling capacity. It could be expected that the neuron would not behave in exactly the same way on every occasion, even if the pattern of inputs was repeated exactly each time. This flexibility and adaptability in neuronal behaviour would impact on the functioning of larger brain units. | 
|
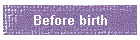
|